Energy, Temperature, and Heat
Energy is the ability or capacity to do work on some form of matter. The potential energy (PE) of any object is given as
PE= mgh
Where m is the object’s mass, g is the acceleration of gravity, and h is the object’s height above the surface. Any moving substance possesses energy of motion, or kinetic energy. The kinetic energy (KE) of an object is equal to half its mass multiplied by its velocity squared; thus KE =1/2MV2 Kinetic energy also depends on the object’s mass, a volume of water and an equal volume of air may be moving at the same speed, but, because the water has greater mass, it has more kinetic energy. The atoms and molecules that comprise all matter have kinetic energy due to their motion. This form of kinetic energy is often referred to as heat energy. Temperature is a measure of the average speed (average motion) of the atoms and molecules, where higher temperatures correspond to faster average speeds.
Suppose we warm a certain volume of air, then the molecules will gain additional energy that will result in increase in average speed of the molecules. At the same time the molecules will also move slightly farther apart due to high velocity- the air becomes less dense. Similarly, if we cool the air then the speed of the air molecules will decrease and air will become denser. Therefore, warm air is less dense and cold air is denser.
Heat is energy in the process of being transferred from one object to another because of the temperature difference between them.
TEMPERATURE SCALES
The air slowly cools, its atoms and molecules would move more and more slowly until the air reaches a temperature of -273°C (4590 F), which is the lowest temperature possible. At this temperature, called absolute zero, the atoms and molecules would possess a minimum amount of energy and theoretically no thermal motion. Absolute zero is the starting point for a temperature scale called the absolute scale, or Kelvin scale.
The Fahrenheit scale was developed in the early eighteenth century by physicist G. Daniel Fahrenheit (1686–1736), who assigned the number 32 to the temperature at which water freezes, and the number 212 to the temperature at which water boils.
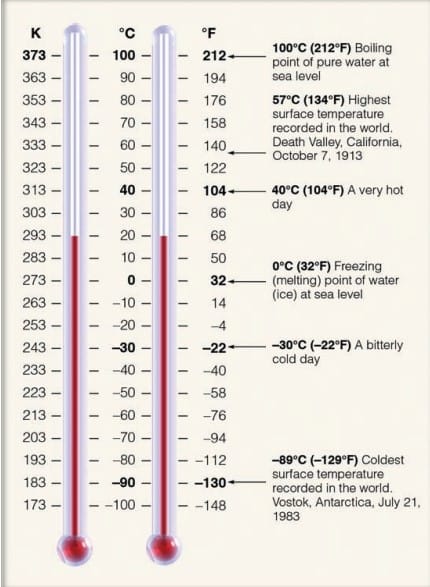
The Celsius scale, named after Swedish astronomer Anders Celsius (1701–1744), was introduced later in the eighteenth century and is part of the metric system used around the world. The number 0 (zero) on this scale is assigned to the temperature at which pure water freezes, and the number 100 to the temperature at which pure water boils at sea level. The space between freezing and boiling is divided into 100 equal degrees. Therefore, each Celsius degree is 180/100 or 1.8 times larger than a Fahrenheit degree.
SPECIFIC HEAT
The heat capacity of a substance is the ratio of the amount of heat energy absorbed by that substance to its corresponding temperature rise. The heat capacity of a substance per unit mass is called specific heat.
SUBSTANCE | SPECIFIC HEAT (cal/g °C) | SPECIFIC HEAT (J/kg °C) |
Water (pure) | 1 | 4186 |
Wet mud | 0.6 | 2512 |
Ice (0°C) | 0.5 | 2093 |
Sandy clay | 0.33 | 1381 |
Dry air (sea level) | 0.24 | 1005 |
Quartz sand | 0.19 | 795 |
Granite | 0.19 | 794 |
Latent (Hidden) Heat
Water vapor is an invisible gas that becomes visible when it changes into larger liquid or solid (ice) particles. This process of transformation is known as a change of state or, simply, a phase change. The heat energy required to change a substance, such as water, from one state to another is called latent heat. If we microscopically examine a water drop, then we can notice that at drop’s surface molecules are constantly evaporating. As faster moving molecules leave the drop easily, so the average speed of the left behind molecules decreases. As we know that the temperature is a measurement of average speed of the molecules, so the slower average motion indicates a lower temperature. Evaporation is, therefore, a cooling process.
The energy needed to evaporate the water—that is, to change its phase from a liquid to a gas—may come from the water or other sources, including the air
The energy lost by liquid water during evaporation can be thought of as carried away by, and “locked up” within, the water vapor molecule. The energy is thus in a “stored” or “hidden” condition and is, therefore, called latent heat. The heat energy will reappear as sensible heat (the heat we can feel, “sense,” and measure with a thermometer) when the vapor condenses back into liquid water. Therefore, condensation (the opposite of evaporation) is a warming process.
The heat energy released when water vapor condenses to form liquid droplets is called latent heat of condensation. Conversely, the heat energy used to change liquid into vapor at the same temperature is called latent heat of evaporation (vaporization). Nearly 600 cal (2500 J) are required to evaporate a single gram of water at room temperature.
latent heat is responsible for keeping a cold drink with ice colder than one without ice. As ice melts, its temperature does not change. The reason for this fact is that the heat added to the ice only breaks down the rigid crystal pattern, changing the ice to a liquid without changing its temperature. The energy used in this process is called latent heat of fusion (melting). Roughly 80 cal (335 J) are required to melt a single gram of ice
The heat energy required to change ice into vapor (a process called sublimation) is referred to as latent heat of sublimation. For a single gram of ice to transform completely into vapor at 00 C requires nearly 680 cal—80 cal for the latent heat of fusion plus 600 cal for the latent heat of evaporation.
Latent heat is an important source of atmospheric energy.
Evaporation–Transportation– Condensation is an extremely important mechanism for the relocation of heat energy (as well as water) in the atmosphere.
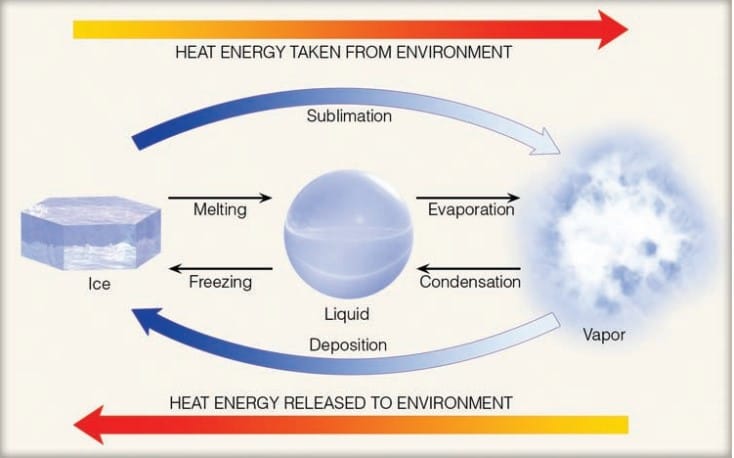
Figure depicts the heat energy released or absorbed during state change of water molecules. When the state change is from left to right i.e., from solid to liquid/ liquid to gas/ solid to gas, heat energy is taken from the environments that results in cooling of the environment. But when state change is from right to left i.e., gas to liquid/ liquid to solid/ gas to solid, heat energy is released to the environment resulting in warming of the surrounding.
Mechanisms of heat transfer
Transfer of heat from molecule to molecule within a substance is called conduction. Greater the temperature difference, more rapid is the heat transfer. The efficiency of a substance to conduct heat depends on the structural bonding of the object. Strongly bonded objects are better conductor of heat. Air is an extremely bad conductor of heat.
The transfer of heat by mass movement of fluid (water or air) s called convection. Convection happens naturally in atmosphere. During sunny and warm day certain areas of the earth heats up more; as result, the air near the surface is heated somewhat unevenly. Air molecules adjacent to these hot surfaces gain extra heat by conduction.
This heated air expands and becomes less dense than the surrounding air. Due to buoyancy this warm air rises and transfer the heat upward. Cooler heavier air flows towards the surface top replace the rising air. This cooler air heats up, rises and cycle repeats again. This vertical exchange of the heat is called convection and the rising air bubbles are known as thermals.
This rising air expands and cools; sinking air compressed and warms. The horizontally moving part of the circulation (called wind) carries properties of the air in that particular area with it. The transfer of these properties by horizontally moving air is called advection. Transfer of energy from one object to another without any medium (in vacuum) is called advection.
Radiant Energy
The energy transferred from the sun to your face is called radiant energy, or radiation. It travels in the form of waves that release energy when they are absorbed by an object. Because these waves have magnetic and electrical properties, we call them electromagnetic waves. In a vacuum, they travel at a constant speed of nearly 300,000 km (186,000 mi) per second—the speed of light. Wavelength of these electromagnetic waves is measured in micrometer (μm).
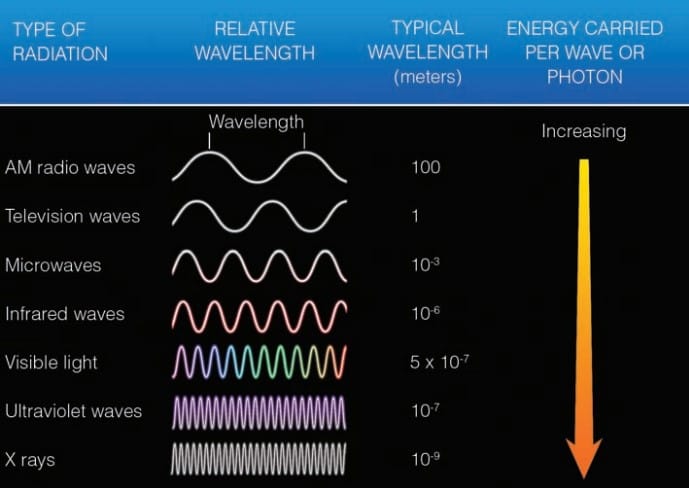
The average wavelength of radiation we can see (called visible light) is about 0.0000005 m, which is the same as 0.5 µm.
RADIATION AND TEMPERATURE
All things (whose temperature is above absolute zero), no matter how big or small, emit radiation. This book, your body, flowers, trees, air, Earth, and the stars are all radiating a wide range of electromagnetic waves. The energy originates from rapidly vibrating electrons, billions of which exist in every object.
Objects that have a very high temperature emit energy at a greater rate or intensity than objects at a lower temperature. Thus, as the temperature of an object increases, more total radiation is emitted each second. This can be expressed mathematically as
E = σT⁴
where E is the maximum rate of radiation emitted by each square meter of surface area of the object, σ (the Greek letter sigma) is the Stefan-Boltzmann constant, and T is the object’s surface temperature in kelvins. This relationship, called the Stefan Boltzmann law.
RADIATION OF THE SUN AND EARTH
The sun’s energy is emitted from its surface, where the temperature is nearly 6000 K (10,500oF)
So, solar radiation is also called shortwave radiation. Whereas, the Earth with an average temperature of 15°C emits almost all of its radiation in IR wavelength and it is referred to as long wave/terrestrial radiation.
The sun and Earth both have characteristics (discussed in a later section) that enable us to use the following relationship called Wien’s law (or Wien’s displacement law) after the German physicist Wilhelm Wien (pronounced Ween, 1864–1928), who discovered it
λₘₐₓ = constant / T
where is the wavelength in micrometers at which maximum radiation emission occurs, T is the object’s temperature in kelvins, and the constant is 2,897 µm K.
Earth emits most of its radiation at longer wavelengths between about 5 and 25 µm. Earth’s radiation (terrestrial radiation) is often called longwave radiation, whereas the sun’s energy (solar radiation) is referred to as shortwave radiation. If we look at the amount of radiation given off by the sun at each wavelength, we obtain the sun’s electromagnetic spectrum. This portion of the spectrum is referred to as the visible region, and the radiant energy that reaches our eye is called visible light. The sun emits nearly 44 percent of its radiation in this zone, with the peak of energy output found at the wavelength corresponding to the color blue-green.
Wavelengths shorter than violet (0.4 µm) are ultraviolet (UV). X-rays and gamma rays with exceedingly short wavelengths also fall into this category. The sun emits only about 7 percent of its total energy at ultraviolet wavelengths. The longest wavelengths of visible light correspond to the color red. Wavelengths longer than red (0.7 µm) are infrared (IR). These waves cannot be seen by the unaided human eye.
Nearly 37 percent of the sun’s energy is radiated between 0.7 µm and 1.5 µm, with only 12 percent radiated at wavelengths longer than 1.5 µm.
The sun and Earth emit radiation. The hot sun (6000 K) radiates nearly 88 percent of its energy at wavelengths less than 1.5 µm, with maximum emission in the visible region near 0.5 µm.
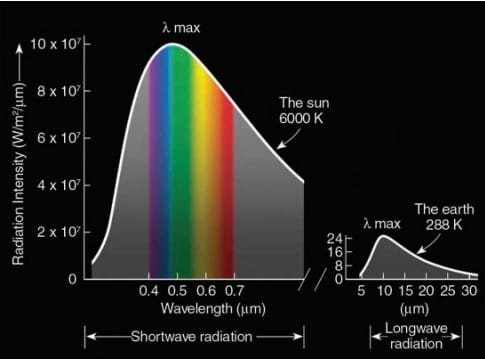
The cooler Earth (288 K) radiates nearly all its energy between 5 and 25 µm with a peak intensity in the infrared region near 10 µm. The sun’s surface is nearly 20 times hotter than Earth’s surface.
Radiation: Absorption, Emission, and Equilibrium
Any object that is a perfect absorber (that is, absorbs all the radiation that strikes it) and a perfect emitter (emits the maximum radiation possible at its given temperature) is called a blackbody. Blackbodies do not have to be colored black; they simply must absorb and emit all possible radiation.
Due to rotation of earth, half of it is in sunlight absorbing solar radiation, whereas, half of it is in darkness emitting IR radiation. If there is no other method of heat transfer, then the rate of emission of IR radiation is equal to the rate of absorption of solar radiation without any change in average temperature. Such condition is called radiative equilibrium condition. The temperature at which the earth receives solar radiation and emits IR radiation is called radiative equilibrium temperature. The radiative equilibrium temperature of the earth is -18°C (255K) which is less than the average temperature of the earth i.e., 15°C. But this temperature is much lower than Earth’s observed average surface temperature of 288K (15oC, 59o F).
Why is there such a large difference???
Earth’s atmosphere absorbs and emits infrared radiation. Unlike Earth, the atmosphere does not behave like a blackbody, as it absorbs some wavelengths of radiation and is transparent to others. So, without atmosphere, the average temperature of earth would be 33°C lower than the present value.
Unlike earth, the atmosphere is not a blackbody. It selectively absorbs only some wavelength of radiation and is transparent to other. Objects that selectively absorb and emit radiation are called selective absorbers. Snow is a good absorber of IR radiation but poor absorber of solar radiation. Objects that selectively absorb radiation usually selectively emit radiation at same wavelength. Snow is therefore a good emitter of IR energy. At night snow emits more IR radiation than it absorbs from surrounding. This large loss of IR energy causes the air above a snow surface on a clear winter night to become extremely cold.
Selective absorbers in atmosphere and greenhouse effect:
Objects that selectively absorb radiation also selectively emit radiation at the same wavelength. This phenomenon is called Kirchhoff ’s law. This law states that good absorbers are good emitters at a particular wavelength, and poor absorbers are poor emitters at the same wavelength.
Snow is a good absorber as well as a good emitter of infrared energy (white snow actually behaves as a blackbody in the infrared wavelengths).
Water vapor (H2O) and carbon dioxide (CO2) are strong absorbers of infrared radiation and poor absorbers of visible solar radiation. Other, less important, selective absorbers include nitrous oxide (N2O) and methane (CH4), as well as ozone (O3), which is most abundant in the stratosphere. As these gases absorb infrared radiation emitted from Earth’s surface, they gain kinetic energy (energy of motion). The gas molecules share this energy by colliding with neighboring air molecules, such as oxygen and nitrogen (both of which are poor absorbers of infrared energy).
Most of the infrared energy emitted from Earth’s surface keeps the lower atmosphere warm. Besides being selective absorbers, water vapor and CO2 selectively emit radiation at infrared wavelengths. A portion of this energy is radiated toward Earth’s surface and absorbed, thus heating the ground. Earth, in turn, constantly radiates infrared energy upward, where it is absorbed and warms the lower atmosphere. Water vapor and CO2 absorb and radiate infrared energy and act as an insulating layer around Earth, keeping part of Earth’s infrared radiation from escaping rapidly into space.
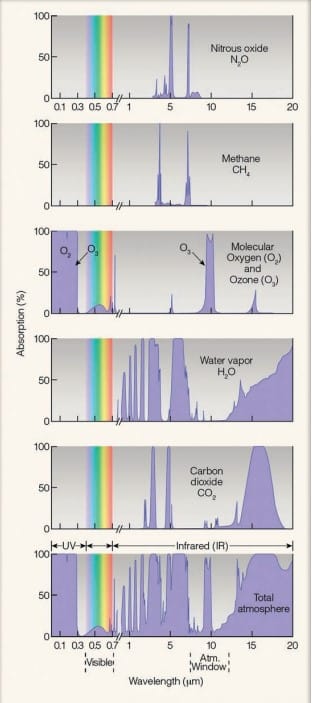
Earth’s mean radiative equilibrium temperature without CO2 and water vapor would be around -18o C (59oF ), or about 33o C (59o F) lower than at present.
The absorption of infrared radiation from Earth by water vapor and CO2 is popularly called the greenhouse effect
The warm air inside a greenhouse is probably caused more by the air’s inability to circulate and mix with the cooler outside air than by the entrapment of infrared energy. Because of these findings. The greenhouse effect should be called the atmosphere effect. To accommodate everyone, we will usually use the term atmospheric greenhouse effect when describing the role that water vapor, CO2, and other greenhouse gases.
Thus, most of the IR energy emitted from earth’s surface keeps the lower atmosphere warm. For this reason, H2O and CO2, CH4, N2O, O3 are called greenhouse gasses. Within a wavelength range of 8-11 μm region neither H2O nor CO2 absorbs IR radiation. So, this wavelength range is called atmospheric window.
Clouds even absorb the wavelengths between 8 and 11 µm, which are otherwise “passed up” by water vapor and CO2. Thus, they have the effect of enhancing the atmospheric greenhouse effect by closing the atmospheric window.
Bases of the clouds radiate IR energy back to the earth surface. This process keeps the calm, cloudy night relatively warmer than the calm, clear ones. During day time the top of the cloud reflects the sunlight. Since the ground does not heat up as much as it would in full sunshine, so cloudy, calm days are cooler than clear, calm days. So, the presence of clouds tends to keep night time temperature higher and day time temperature lower.
Apart from cloud, CFC-12 absorbs the radiation in a region of 8-11μm region. Addition of one molecule of CFC is equivalent to the addition of 10,000 CO2 molecules in terms of heat absorption. Overall, water vapor accounts for 60%, CO2 for 26% and remaining gasses for 14% of the atmospheric greenhouse effects.
Warming of the air from below
Solar energy warms the earth’s surface without heating the surrounding air. The air molecules colliding with this heated surface gets warmed by conduction, also, they transfer the heat to neighboring molecules.
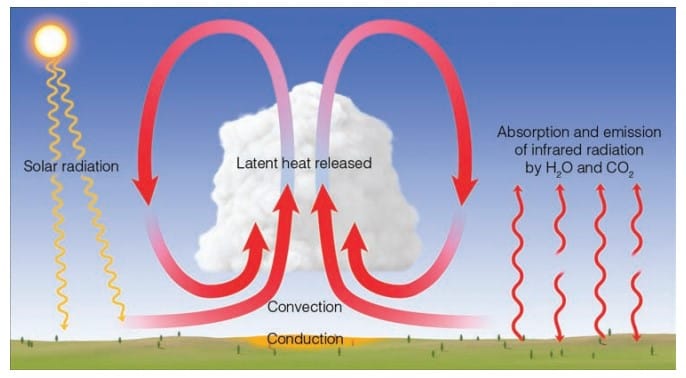
But this process is only important within a few centimeters of the ground due to bad conductivity of air. As the surface air warms it becomes less dense than the air directly above it. The warmer air rises setting up thermals/ free convection cells, that distribute the heat through the deeper layer of air. The rising air expands and cools; the water vapor in it condenses into cloud droplets, releasing latent heat, that warms the air. At the same time, the outgoing IR radiation by earth is absorbed by the greenhouse gasses. As the concentration of the water vapor decreases rapidly above the earth’s surface, so most of the IR energy is absorbed near the earth’s surface. Hence, the lower atmosphere is heated from below.
Incoming solar Energy
At the top of the atmosphere, solar energy received on a surface perpendicular to the sun’s ray appear remains constant at two calories on each cm2 each minute or 1361W/m2– a value called the solar constant. [the solar constant (which, in actuality, is not “constant”) is the rate at which radiant energy from the sun is received on a surface at the outer edge of the atmosphere perpendicular to the sun’s rays when Earth is at an average distance from the sun.]
Solar constant on any planet
Sₒ = T⁴ × (R/d)²
Where T= Temperature of the sun, R= Radius of the sun, d= distance between the sun and the planet.
When solar radiation enters into the atmosphere, some energy is absorbed by the greenhouse gasses, some are scattered and some are scattered. As air molecules are much smaller that the wavelength of sunlight, so they scatter the blue light most. As scattered blue light comes into our eyes from every direction that’s why sky is blue.
The distribution of light in this manner is called scattering. (Scattered light is also called diffuse light.)
If the size of the air molecules were greater than that of the wavelength of visible light then they would scatter red light. During sunrise and sunset, the sun light has to travel through a thicker layer of the atmosphere, scattering by air molecules removes the blue light causing the sun to be appear as red. During noon, all the visible light from the sun strikes our eyes and the sun is perceived as white. Sunlight can also reflect from objects after striking on it.
The percent of solar radiation that reflects from an object after striking on it is called Albedo of an object.
SURFACE | ALBEDO (PERCENT) |
Fresh snow | 75 to 95 |
Clouds (thick) | 60 to 90 |
Clouds (thin) | 30 to 50 |
Venus | 78 |
Ice | 30 to 40 |
Sand | 15 to 45 |
Earth and atmosphere | 30 |
Mars | 17 |
Grassy field | 10 to 30 |
Dry, plowed field | 5 to 20 |
Water | 10 |
Forest | 3 to 10 |
Moon | 7 |
Cloud is the largest contributor of planetary albedo. Planet Venus has an albedo of 78%, due to presence of dry ice (solid CO2) in its atmosphere. Earth and its atmosphere have an albedo of 30%.
When solar wind interacts with earth’s atmosphere, a dazzling light called Aurora generates in high latitudes. Due to high temperature of the sun, the gasses present in the sun becomes stripped of electrons and capable of escaping the sun’s gravitational field. These high velocities charged particles are called solar wind.
When solar wind moves close to the earth, it interacts with the earth’s magnetic field and disturb it. Due to this disturbance some energetic solar wind enters into the upper atmosphere, where they collide with atmospheric gasses. These gasses become excited and emit visible radiation, which causes the sky to glow like a neon light-producing Aurora. Aurora in N. Hemisphere is called Aurora Borealis and in S. Hemisphere is called Aurora Australis.
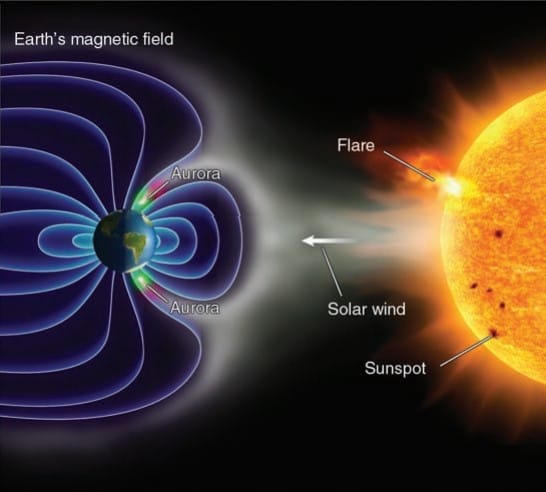