Rare Earth Elements (REE)
The rare earth elements (REE) are the most useful of all trace elements, with significant applications in igneous, sedimentary, and metamorphic petrology. The REE comprise the series of metals with atomic numbers 57 to 71 (La to Lu). Additionally, the element Y, which has an ionic radius similar to that of the REE Ho, is sometimes included in this group. Typically, the low-atomic-number members of the series are termed the light rare earths (LREE), while those with higher atomic numbers are known as heavy rare earths (HREE). Less commonly, the middle members of the group, Sm to Ho, are referred to as the middle REE (MREE).
Table 1: The Rare Earth Elements
Atomic Number | Name | Symbol | Ionic Radius for Eight-Fold Coordination * |
---|---|---|---|
57 | Lanthanum | La | La³⁺ 1.160 |
58 | Cerium | Ce | Ce³⁺ 1.143 Ce⁴⁺ 0.970 |
59 | Praesodymium | Pr | Pr³⁺ 1.126 |
60 | Neodymium | Nd | Nd³⁺ 1.109 |
61 | Promethium | Pm | Not naturally occurring |
62 | Samarium | Sm | Sm³⁺ 1.079 |
63 | Europium | Eu | Eu³⁺ 1.066 Eu²⁺ 1.250 |
64 | Gadolinium | Gd | Gd³⁺ 1.053 |
65 | Terbium | Tb | Tb³⁺ 1.040 |
66 | Dysprosium | Dy | Dy³⁺ 1.027 |
67 | Holmium | Ho | Ho³⁺ 1.015 |
68 | Erbium | Er | Er³⁺ 1.004 |
69 | Thulium | Tm | Tm³⁺ 0.994 |
70 | Ytterbium | Yb | Yb³⁺ 0.985 |
71 | Lutetium | Lu | Lu³⁺ 0.977 |
39 | Yttrium | Y | Y³⁺ 1.019 |
* From Shannon (1976), in Ångstroms (10⁻¹⁰ m).
The Chemistry of the REE
All REE share very similar chemical and physical properties due to their ability to form stable 3+ ions of similar size. The slight differences in chemical behavior arise from the small but steady decrease in ionic size with increasing atomic number. These small differences are exploited by various petrological processes, causing the REE series to become fractionated relative to each other. This phenomenon is used in geochemistry to explore the genesis of rock suites and to unravel petrological processes.
While a small number of REE also exist in oxidation states other than 3+, the only ions of geological importance are Ce4+ and Eu2+. These ions form a smaller and a larger ion, respectively, relative to the 3+ oxidation state.
Presenting REE Data
Rare earth element concentrations in rocks are usually normalized to a common reference standard, most commonly the values for chondritic meteorites. Chondritic meteorites were chosen because they are believed to be relatively unfractionated samples of the solar system dating from the original nucleosynthesis. However, REE concentrations in the solar system are highly variable due to the different stabilities of atomic nuclei. REE with even atomic numbers are more stable and, therefore, more abundant than REE with odd atomic numbers, producing a zig-zag pattern on a composition-abundance diagram. This pattern of abundances is also found in natural samples.
Chondritic normalization serves two important functions. First, it eliminates the abundance variation between odd and even atomic number elements. Second, it allows any fractionation of the REE group relative to chondritic meteorites to be identified. Normalized values and ratios of normalized values are denoted with the subscript N—hence, for example, CeN and (La/Ce)N.
The REE are typically presented on a concentration vs. atomic number diagram where concentrations are normalized to the chondritic reference value and expressed as the logarithm to the base 10 of the value. Concentrations at individual points on the graph are joined by straight lines. This is sometimes referred to as the Masuda-Coryell diagram, named after the original proponents of the diagram (Masuda, 1962; Coryell et al., 1963). Trends on REE diagrams are usually referred to as REE “patterns,” and the shape of an REE pattern is of considerable petrological interest.
Not infrequently, the plotted position of Eu lies off the general trend defined by the other elements on an REE diagram, potentially defining a europium anomaly. If the plotted composition lies above the general trend, the anomaly is described as positive; if it lies below the trend, it is considered negative. Europium anomalies can be quantified by comparing the measured concentration (Eu) with an expected concentration obtained by interpolating between the normalized values of Sm and Gd (Eu*). Thus, the ratio Eu/Eu* is a measure of the europium anomaly, and a value greater than 1.0 indicates a positive anomaly, while a value less than 1.0 indicates a negative anomaly.
Difficulties with Chondrite Normalization
Unfortunately, it has become apparent that chondritic meteorites are quite variable in composition, with “chondrites with ‘chondritic’ REE abundances being the exception rather than the rule” (Boynton, 1984). This variability in chondritic composition has given rise to a large number of sets of normalizing values for the REE, and to date, no standardized value has been adopted. The variability can be attributed to two factors: the analytical method and the precise type of chondrites analyzed. Some authors use “average chondrite,” while others select Cl chondrites as the most representative of the original solar nebula’s composition.
Choosing a Set of Normalizing Values
Figure 1 shows flat rare earth patterns typical of an Archaean tholeiite normalized to the range of chondritic values listed in Table 2. The patterns display both variety in shape and concentration range. The consensus seems to favor values based on average chondrite rather than Cl chondrites, and either the values of Boynton (1984), based on Evensen et al. (1978), or the values of Nakamura (1974), with additions from Haskin et al. (1968), appear satisfactory. In fact, the two sets of values are very similar and lie in the middle of the range of values currently in use.
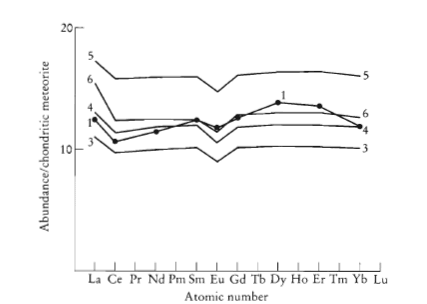
Table 2 : Chondrite Values Used in Normalizing REE (Concentrations in ppm)
(Ref.) | La | Ce | Pr | Nd | Sm | Eu | Gd | Tb | Dy | Ho | Er | Tm | Yb | Lu | Y |
---|---|---|---|---|---|---|---|---|---|---|---|---|---|---|---|
Wakita 1 | 0.340 | 0.910 | 0.121 | 0.640 | 0.195 | 0.073 | 0.260 | 0.047 | 0.300 | 0.078 | 0.020 | 0.032 | 0.220 | 0.034 | |
Haskin 2 | 0.330 | 0.880 | 0.112 | 0.600 | 0.181 | 0.069 | 0.249 | 0.047 | 0.300 | 0.070 | 0.200 | 0.030 | 0.200 | 0.034 | |
Masuda 3 | 0.378 | 0.976 | 0.716 | 0.716 | 0.230 | 0.086 | 0.311 | 0.390 | 0.390 | 0.070 | 0.255 | 0.249 | 0.249 | 0.038 | |
Nakamura 4 | 0.329 | 0.865 | 0.630 | 0.630 | 0.203 | 0.077 | 0.276 | 0.343 | 0.343 | 0.071 | 0.225 | 0.220 | 0.220 | 0.033 | |
Evensen 5 | 0.244 | 0.637 | 0.096 | 0.473 | 0.154 | 0.058 | 0.204 | 0.254 | 0.254 | 0.056 | 0.176 | 0.171 | 0.171 | 0.026 | |
Anders 6 | 0.336 | 0.891 | 0.113 | 0.619 | 0.186 | 0.071 | 0.256 | 0.046 | 0.306 | 0.071 | 0.202 | 0.031 | 0.202 | 0.032 | |
Asuka 7 | 0.338 | 0.899 | 0.132 | 0.627 | 0.188 | 0.072 | 0.259 | 0.046 | 0.309 | 0.073 | 0.206 | 0.032 | 0.206 | 0.032 | |
Gascoigne 8 | 0.340 | 0.905 | 0.122 | 0.633 | 0.191 | 0.073 | 0.263 | 0.047 | 0.313 | 0.074 | 0.211 | 0.033 | 0.211 | 0.033 | |
Mason 9 | 0.341 | 0.906 | 0.123 | 0.635 | 0.193 | 0.073 | 0.264 | 0.047 | 0.315 | 0.074 | 0.212 | 0.033 | 0.212 | 0.033 |
REE Ratio Diagrams
The degree of fractionation of an REE pattern can be expressed by the concentration of a light REE (La or Ce) ratioed to the concentration of a heavy REE (Yb or Y). Both elements are chondrite-normalized. The ratio (La/Yb)N is often plotted against either CeN or YbN on a bivariate graph and serves as a measure of the degree of REE fractionation with changing REE content. Similar diagrams can be constructed to measure the degree of light REE fractionation [(La/Sm)N vs. SmN], heavy REE fractionation [(Gd/Yb)N vs. YbN], and Eu anomaly [(La/Sm)N vs. (Eu/Eu*)] in individual REE patterns.
NASC Normalization for Sediments
It has been observed that the concentration of many elements in fine-grained sedimentary rocks on continental platforms worldwide is similar due to mixing through repeated cycles of erosion. This “average sediment” is often used as the normalizing value for REE concentrations in sedimentary rocks. A frequently used composition is that of the North American Shale Composite (NASC), and the recommended values of Gromet et al. (1984) are provided in Table 3 (column 5). Alternatives to NASC in current use include a composite European shale (Haskin and Haskin, 1966) and the post-Archaean average Australian sedimentary rock (McLennan, 1989). Some authors consider the average abundance of REE in sedimentary rocks as a measure of the REE content of the upper continental crust, assuming that sedimentary processes homogenize the REE previously fractionated during the formation of igneous rocks. Thus, an alternative to shale normalization is to use values for the average upper continental crust (Table 3, column 8).
Table 3: Standard Sedimentary Compositions Used for Normalizing the REE Concentrations in Sedimentary Rocks (Concentrations in ppm)
(Ref.) | La | Ce | Pr | Nd | Sm | Eu | Gd | Tb | Dy | Ho | Er | Tm | Yb | Lu | Y |
---|---|---|---|---|---|---|---|---|---|---|---|---|---|---|---|
NASC 1 | 39.00 | 76.00 | 10.30 | 37.00 | 7.00 | 2.00 | 6.10 | 1.30 | 4.00 | 1.40 | 4.00 | 0.58 | 3.40 | 0.60 | 27.00 |
NASC 2 | 32.00 | 70.00 | 7.90 | 31.00 | 5.70 | 1.24 | 5.21 | 0.85 | 3.40 | 1.04 | 3.40 | 0.50 | 3.10 | 0.48 | |
NASC 3 | 32.00 | 73.00 | 7.90 | 33.00 | 5.70 | 1.24 | 5.20 | 0.85 | 3.40 | 1.04 | 3.40 | 0.50 | 3.10 | 0.48 | |
NASC 4 | 31.10 | 66.70 | 10.40 | 27.40 | 5.59 | 1.18 | 5.50 | 0.85 | 1.04 | 1.04 | 3.40 | 0.50 | 3.10 | 0.48 | |
NASC 5 | 31.10 | 67.03 | 10.40 | 30.40 | 5.98 | 1.25 | 5.50 | 0.85 | 1.04 | 1.04 | 3.40 | 0.50 | 3.11 | 0.46 | |
ES | 41.10 | 81.30 | 10.40 | 40.10 | 7.30 | 1.52 | 6.03 | 1.05 | 1.20 | 1.20 | 3.55 | 0.56 | 3.29 | 0.58 | |
PAAS | 38.20 | 79.60 | 8.83 | 33.90 | 5.55 | 1.08 | 6.03 | 0.77 | 4.68 | 1.20 | 3.50 | 0.56 | 2.82 | 0.43 | 31.80 |
Upper crust | 30.00 | 64.00 | 7.10 | 26.00 | 4.50 | 0.88 | 4.66 | 0.77 | 3.50 | 0.80 | 2.30 | 0.33 | 2.20 | 0.32 | 27.00 |
Typical seawater | 20.80 | 9.60 | 8.83 | 21.10 | 4.32 | 0.88 | 3.80 | 0.64 | 3.80 | 0.88 | 2.30 | 0.33 | 2.20 | 0.32 | 27.00 |
River water | 425.0 | 6.60 | 365.0 | 80.4 | 80.4 | 2.70 | 83.5 | 7.80 | 97.9 | 6.40 | 64.6 | 3.30 | 51.7 | 3.20 | 22.00 |
References:
- North American shale composite (Haskin and Frey, 1966).
- North American shale composite (Haskin and Haskin, 1966).
- North American shale composite (Haskin et al., 1968).
- North American shale composite (Gromet et al., 1984) — INAA.
- North American shale composite (Gromet et al., 1984) — recommended.
- Average European shale (Haskin and Haskin, 1966).
- Post-Archaean average Australian sedimentary rock (McLennan, 1989).
- Average upper continental crust (Taylor and McLennan, 1985).
- Elderfield and Greaves (1982), Table 10, p. 51.
- Hoyle et al. (1984): River Luce, Scotland, estuary.
Relative to chondritic meteorites, NASC has about 100 times the light REE content, about 10 times the heavy REE content, and a small negative Eu anomaly. Normalization against NASC serves as a measure of how typical a sediment is and can identify subtle enrichments and deficiencies in certain elements.
Rock Normalization
Less commonly, some authors normalize REE concentrations to a particular sample in a rock suite as a measure of relative change. This approach is also useful when the REE concentrations of the individual minerals in the rock have been determined, allowing them to be expressed relative to the concentration in the whole rock. A similar form of normalization is to express the concentration in a mineral relative to the composition of the groundmass; this is frequently used to display mineral/melt partition coefficients.
Interpreting REE Patterns
The REE are considered among the least soluble trace elements and are relatively immobile during low-grade metamorphism, weathering, and hydrothermal alteration. Michard (1989) demonstrates that hydrothermal solutions contain significantly fewer REE than the reservoir rock through which they pass, indicating that hydrothermal activity is not expected to have a major effect on rock chemistry unless the water/rock ratio is very high. However, the REE are not entirely immobile, as emphasized in the review by Humphries (1984), and caution should be exercised when interpreting the REE patterns of heavily altered or highly metamorphosed rocks. Nevertheless, REE patterns in slightly altered rocks can faithfully represent the original composition of the unaltered parent, and a fair degree of confidence can be placed in the significance of peaks, troughs, and the slope of an REE pattern.
REE Patterns in Igneous Rocks
The REE pattern of an igneous rock is controlled by the REE chemistry of its source and the crystal-melt equilibria that have occurred during its evolution. Here, we describe qualitatively how the roles of individual minerals may be identified during magmatic evolution, either during partial melting in the source or fractional crystallization in the magma chamber. If equilibrium (as opposed to fractional) melting or crystallization takes place, the concentration of REE in a melt is determined by the bulk distribution coefficient of the REE between melt and solid. The bulk distribution coefficient depends on the proportions of minerals in the residue or the crystallizing assemblage and the individual partition coefficients of the minerals.
REE Partitioning
The distribution coefficients of the REE for the most common rock-forming minerals are shown in Table 4.7. The high-field-strength cations, particularly the REE, do not substitute readily into most rock-forming minerals. Consequently, when equilibrium melting or crystallization takes place, the bulk distribution coefficients for these elements are typically much less than 1.0. This implies that melting produces large degrees of REE fractionation, but crystallization produces relatively minor fractionation. Additionally, the higher the degree of partial melting, the smaller the degree of REE fractionation in the melt, as there is a decreasing difference between the proportion of REE in the melt and solid phases.
REE Partition Coefficients
The REE readily substitute into minerals such as garnet, epidote, amphibole, and apatite, all of which have relatively high partition coefficients. If any of these minerals is present in the residue of partial melting or as an early crystallizing phase, the pattern of REE fractionation is modified accordingly.
For instance, if garnet is present in the residue, then the heavy REE, such as Yb, are preferentially retained in the residue, producing melts with depleted heavy REE contents and a steep positive slope on a chondrite-normalized REE diagram. Alternatively, if a garnet-bearing melt undergoes fractionation with no other REE-hosting phase, then the opposite effect occurs, and the melt becomes progressively enriched in heavy REE. The presence of garnet in the residue of a melt can therefore be inferred if the REE pattern of the melt shows a pronounced positive slope, which is indicative of heavy REE depletion.
Similarly, if apatite is a crystallizing phase, the REE pattern of the magma is modified to produce negative slopes in both the light and heavy REE and, consequently, a concave upward REE pattern. If a magma shows no heavy REE depletion but displays a negative slope for the light REE, the presence of a light REE-hosting mineral such as apatite or an accessory phase, such as epidote or zircon, can be inferred.
Conclusion
Rare earth elements are an essential tool in modern geochemistry, offering deep insights into the processes governing the formation of rocks. The systematic behavior of REEs allows geologists to infer the history of rock formation, from the original source material to the various melting and crystallization events that shape the final rock composition. Through careful analysis of REE patterns, geochemists can unlock the history stored in the rocks, providing a clearer picture of Earth’s dynamic processes.